Biomolecules
- 90% of the dry weight of the human body is made up of six elements: Carbon (C), Hydrogen (H), Oxygen (O), Nitrogen (N), Phosphorus (P), and Sulfur (S). These six elements make up the majority of biological matter. Carbon is the most pervasive element in existence.
- Other elements found in living things include, among others, calcium (Ca), potassium (K), sodium (Na), chloride (CI), magnesium (Mg), copper (Cu), cobalt (Co), iodine (I), zinc (Zn), fluorine (F), molybdenum (Mo), and selenium (Se).
- Any chemical substance known as an organic compound has carbon bonded covalently to another element, usually hydrogen, oxygen, or nitrogen. These organic chemicals are the building blocks of the most complicated biomolecules. These biomolecules, their component parts, and their roles are summarized in the table..
Major Biomolecules and their functions
Biomolecules |
Building blocks |
Functions |
Carbohydrates
(eg. Glycogen)
|
Monosaccharides
(Glucose)
|
Provide energy and can be stored in animal cells
|
Proteins
(eg. Collagen)
|
Amino acids |
Fundamental basis for structure and functions of cell |
Lipids
(eg. Triglyceride)
|
Fatty acids, glycerol |
Concentrated source of energy to meet long term energy demands |
DNA |
Deoxyribonucleotides |
Transfer hereditary character from parents to offspring |
RNA |
Ribonucleotides |
Required for protein synthesis |
Chemical composition of a norma human being weighing 65 kg
Constituent |
Percent (%) |
Weight (kg) |
Water |
61.6 |
40 |
Protein |
17 |
11 |
Lipid |
13.8 |
9 |
Carbohybrate |
1.5 |
1 |
Minerals |
6.1 |
4 |
Carbohydrates
- The most prevalent organic molecule in nature is a carbohydrate. The term "carbohydrates" literally refers to carbon hydrates. ( H {2}*O .C) The empirical formula for carbohydrates is (C. H 2*O)n or CnH2nOn.
- Poly-hydroxy aldehydes, poly-hydroxy ketones, and any other molecules that break down into these substances during hydrolysis are referred to as carbohydrates. For instance, glucose and fructose. Heparin, glucose, lactose, sucrose, hyaluronic acid, etc.
_1668044816.jpg)
Carbohydrates are categorized Carbohydrates are divided into 3 groups based on the quantity of sugar units they contain:
- Monosachharides (sugar = mono; one = one)
- A few oligosachharides.
- Several polysachharides.
Monosachharides
- These sugars are the most basic.
- They can't undergo any more hydrolysis.
- These taste sweet and are water soluble. such as fructose, glucose, etc.
Classification of Monosaccharides:
On the basis of the number of carbon atoms as well as the presence of an aldehyde or ketone group, monosaccharides can be further categorized.
- They are divided into four categories based on the number of carbon atoms: trioses, tetroses, pentoses, and hexoses.
- They can be categorized as either aldoses (sugars with an aldehyde group, like ribose or glucose) or ketoses depending on whether they contain an aldehyde or ketone group.
The classification of monosaccharides is listed below according to the number of carbon atoms and the presence of an aldehyde or ketone group:
No. of Carbon |
Aldoses |
Ketoses |
3 (Trioses) |
Glyceraldehyde |
Dihydroxyacetone |
4 (Tetroses) |
Erythrose |
Erythrulose |
5 (Pentoses) |
Ribose |
Ribulose |
6 (Hexoses) |
Glucose |
Fructose |
Isomerism in Monosaccharides:
By definition, asymmetric carbon is surrounded by four different groups or atoms. The quantity of asymmetric carbon atoms in a chemical, 2n, which determines its potential isomer. Due to the fact that glucose contains 4 asymmetric carbon atoms, it has 2 4 = 16 isomers. When two substances have the same structural formula but distinct atom configurations within the molecule, they are said to be isomers or stereoisomers. Different characteristics set isomers apart. Here are a few illustrations of the various isomerisms that monosaccharides display:
- D and L isomer
- d and l isomer
- α and β isomer
Oligosaccharides
- When oligosaccharides are hydrolyzed, 2–10 monosaccharides are released.
- These are also sweet compounds that are water soluble.
Disaccharides, trisaccharides, tetrasaccharides, pentasaccharides, etc. are additional categories for oligosaccharides.
- Disaccharides
such as lactose, sucrose, and maltose. When the two monosaccharide units in these sugars are hydrolyzed, the constituent monosaccharides, such as, are created. Upon hydrolysis, maltose yields glucose and glucose.
- Trisachharides
Three monosaccharide units make up these carbohydrates, and when those units are hydrolyzed, the constituent monosaccharides are created. eg. When hydrolyzed, raffinose yields glucose, fructose, and galactose, while maltotriose yields three glucose units.
- Tetrasaccharides
When these carbohydrates are hydrolyzed, four monosaccharide units are released. Stachyose, for instance, releases glucose, fructose, and two galactoses.
- Pentasaccharides
There are five monosaccharide units in this carbohydrate, which are released during hydrolysis. Galactose, glucose, and fructose are released by verbascose, for example.
Polysaccharides
Polysaccharides contain more than ten (>10) monosaccharide units. In other words, the hydrolysis of polysaccharides results in the production of more than ten (11 or so) molecules of monosaccharide. starch, glycogen, hyaluronic acid, heparin, etc. Colloids are created when they interact with water and normally have no taste. They can also be categorized as
- Homopolysaccharides
- Heteropolysaccharides
Homopolysaccharides:
These contain numerous sugar units of a similar type that are released during hydrolysis. For instance, many glucose units can be released from starch, glycogen, cellulose, and inulin, among other substances.
-
Starch
- Glycogen
-
Heteropolysaccharides
- These include several sugar units of various different sorts. Other names for heteropolysaccharides include mucopolysaccharides, heteroglycans, and glycosaminoglycans (GAGs).
- These are composed of uronic acids and aminosugars in repeating units. Additionally, these also include sulphate, carboxylic acid, and acetylated amino groups. for example, chondroitin sulfate, hyaluronic acid, and heparin.
Composition of Carbohydrates
Organic substances known as carbohydrates are mostly made up of the following three elements: carbon (C), hydrogen (H), oxygen (O), etc. Heteropolysaccharides, a type of carbohydrate, also contain certain amino, sulphate, and carboxylic acid groups in addition to these three components.
Sources/Occurrence of Carbohydrates
The sources of carbohydrates are: Cereals (rice, potato, wheat etc.), meat and meat products and milk and milk products etc.
Functions/Importance of Carbohydrates
- Carbohydrates are the chief dietary source of energy. One gram of carbohydrate provides 4kcal or 4 Cal (4 kilo calorie) energy.
- Carbohydrate is one of the components of cell membrane in form of Glycoproteins, Glycolipids, etc.
- Carbohydrates are stored in living beings. Glycogen is stored in animal cells which meet the energy demand of the body. Starch is stored in plant cells which is the major component of dietary carbohydrates.
- Carbohydrates in body may be converted to fats and amino acids.
- Carbohydrate such as heparin acts as an anticoagulant.
- Starch is used in manufacture of paper, textiles etc.
- Carbohydrates form glycosides. Glycosides have medical importance, eg. digitonin is a cardiac stimulant; streptomycin is used in treatment of tuberculosis etc.
Metabolism
- Different types of biomolecules which we take in diet (such as carbohydrates, proteins, lipids, etc.) are digested in gastro-intestinal tract and are converted into the simpler form. For eg. the complex carbohydrate, such as starch is converted to a number of glucose units, proteins may be converted to amino acids, similarly, lipid, such as triglyceride is converted to fatty acids and glycerol.
- Thus produced simpler forms are absorbed from intestine which are transported through blood stream to the cells of various organs where they participate in various chemical reactions.
- The different types of chemical reactions which take place inside a cell in an organized and meaningful manner are collectively called as Metabolism. Literally, metabolism (Greek metabole = change) means a change. Metabolism describes a change in biomolecules during their chemical reactions. The chemical reaction taken up by an individual biomolecule is also called as metabolic pathway.
- Metabolism helps understand :
- The interrelationships of metabolic pathways of different biomolecules,
- Synthesis of lage and complex molecules in the body, and .
- The production of energy such as ATP (Adenosine triphosphate) in the cells.
Metabolism or metabolic pathway fall into three categories
- Anabolic pathway (means, upward or synthesizing change):
- In this type of pathway, large and complex molecules are synthesized from simpler forms.
- The energy required for this process comes from the catabolic pathway. eg. Synthesis of proteins, synthesis of triglyceride, synthesis of glycogen, etc.
- Catabolic pathway (means, degradative change):
- In this pathway, large and complex molecules are degraded into simpler, monomeric forms.
- Energy (ATP) is produced during catabolic pathway.
- Production of energy is in form of high energy phosphate or reducing equivalents.
- Catabolic pathway generally occurs in mitochondria.
- eg. Glycolysis (breakdown of glucose); Proteolysis (breakdown of proteins) and Lipolysis (breakdown of TG)
- Catabolism can be discussed into three stages:
- Primary metabolism: Digestion of large and complex molecules in GIT to form smaller units.
- Secondary or intermediary metabolism: Smaller products are absorbed and are oxidized inside the cell with the liberation of NADH or FADH2 as well as CO2.
- Tertiary metabolism or internal respiration: NADH and FADH2 enter into ETC (electron transport chain) in Mitochodria where oxidation of NADH gives 3 ATP and FADH2 gives 2 ATP. (Note: ATP (Adenosine triphosphate is considered as the energy currency of the cell).
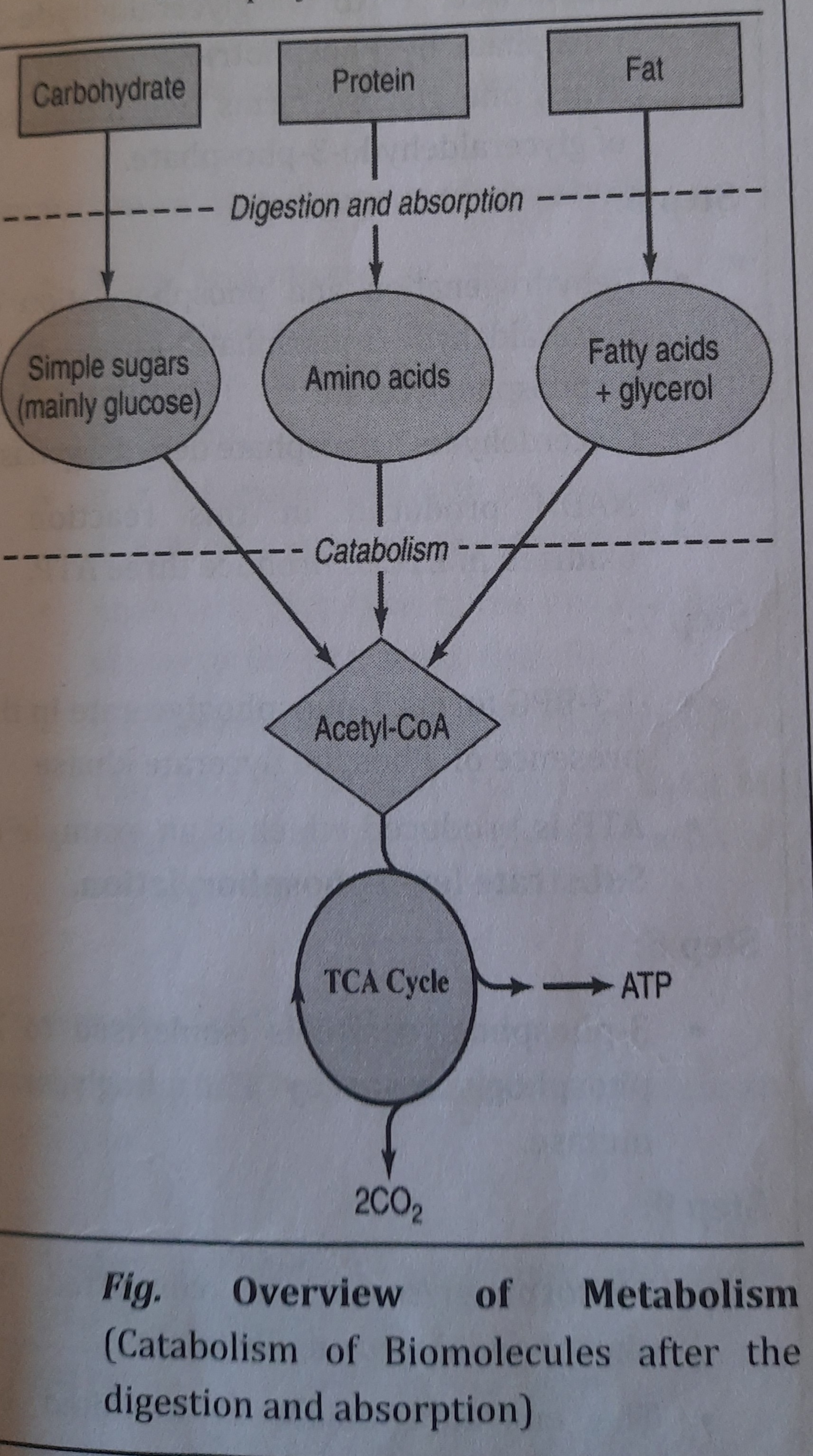
- Amphibolic pathway (Amphi means both; i.e. both type of change):
- This pathway acts as a link between the anabolic and catabolic pathways. In this type of pathway both the anabolism and catabolism occur. eg. Tricarboxylic acid (TCA) cycle
Carbohydrate Metabolism:
1. Glycolysis (Greek, glykys-sweet or glucose or sugar; and lysis= splitting or breakdown)
Definition:
- Glycolysis is defined series of as reactions where glucose is broken down to pyruvate with the production of ATP in aerobic condition. However, under anaerobic condition glucose forms lactate.
- This pathway is also termed as EMP (Embden-Meyerhof-Parnas) pathway after the name of discoverer.
Location: Cytosol or cytoplasm.
- Enzymes required for glycolysis are present in cytosol of all cells, hence this pathway is known as universal pathway.
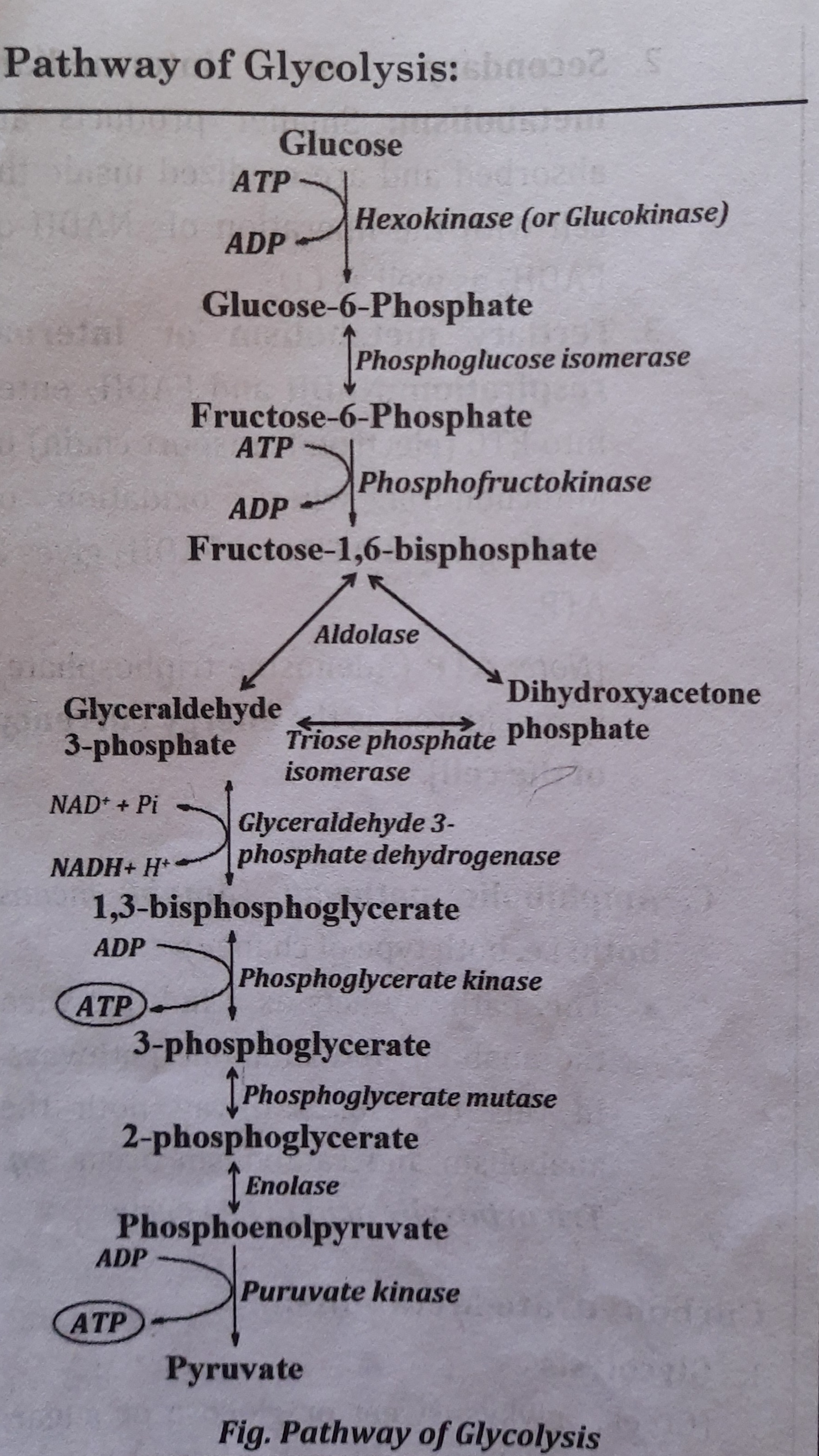
Reactions / pathway of Glycolysis:
- Step 1:
- Glucose is phosphorylated to form Glucose-6-phosphate
- Enzyme involved is Hexokinase or Glucokinase that requires Mg++.
- Step 2:
- Glucose-6-phosphate is isomerized to fructose-6-phosphate by phosphohexose isomerase.
- Step 3:
- Fructose-6-phosphate is again phosphorylated to form fructose-1,6 bisphosphate in the presence of Phosphofructokinase (PFK).
- Step 4:
- Fructose-1,6-bisphosphate is cleaved into glyceraldehyde-3-phosphate and dihydroxyacetone phosphate by Aldolase.
- Step 5:
- Dihydroxyacetone phosphate is isomerised to glyceraldehyde-3 phosphate by Phosphotriose isomerase. Thus, one glucose forms two molecules of glyceraldehyde-3-phosphate.
- Step 6:
- Dehydrogenation and phosphorylation of Glyceraldehyde-3-phosphate forms 1, 3 bisphosphoglycerate (1,3-BPG) by Glycerdehyde-3-phosphate dehydrogenase
- NADH produced in this reaction is oxidized in ETC to produce three ATP.
- Step 7:
- 1,3-BPG forms 3-phosphoglycerate in the presence of Phosphoglycerate kinase • ATP is produced which is an example of Substrate level phosphorylation.
- Step 8:
- 3-phosphoglycerate is isomerised to 2 phosphoglycerate by Phosphoglycerate mutase.
- Step 9:
- 2-phosphoglycerate is converted to phosphoenolpyruvate by Enolase.
- The enzyme Enolase is inhibited by fluorine. [for sugar estimation, blood is collected in sodium fluoride containing vial. Fluorine inhibits enolase and thus stops glycolysis. in the vial. Hence, the value of glucose is not decreased and gives the exact value of glucose in blood even after certain hour of blood withdrawn.]
- Step 10:
- Phosphoenolpyruvate (PEP) is dephosphorylated to the pyruvate in the presence of Pyruvate kinase.
- ATP is generated which is an example of substrate level phosphorylation. [Note: Hexokinase (HK), Phosphofructokinase (PFK) and Pyruvate kinase (PK) are the rate limiting or the key enzymes of glycolysis.]
Importance/ significances of glycolysis
- Main importance of glycolysis is the production of ATP [Net 8 ATP in aerobic glycolysis while 2 ATP in anaerobic glycolysis].
- It is the major pathway for synthesis of ATP in tissues which lack mitochondria.
- Anaerobic glycolysis is the major source of energy for exercising muscles.
- It is the only the source of energy in RBC.
- The intermediates of glycolysis, such as Glucose-6-P is used for the synthesis of Glycogen, Ribose sugar, etc.
Energetics of Glycolysis:
Production of ATPs per molecule of glucose in glycolysis
- Under an aerobic condition, glycolysis produces 8 ATPs
Enzymes |
ATP;
(Utilization (-) or Production (+)
|
Hexokinase |
- 1 ATP |
Phosphofructokinase |
-1 ATP |
Glyceraldehyde-3-phosphate dehydrogenase(2 NADH) |
+6 ATP |
Phosphoglycerate kinase |
+2 ATP |
Pyruvate kinase |
+2 ATP |
Net gain of ATP |
8 ATP |
- Under anaerobic condition or in tissues lacking mitochondria, it produces only 2 ATPs
Enzymes |
ATP;
Utilizatin (-) or Production (+)
|
Hexokinase |
-1 ATP |
Phosphofructokinase |
-1 ATP |
Phosphofructokinase |
+2 ATP |
Pyruvate kinase |
+2 ATP |
Net gainof ATP |
2 ATPs |
Regulation of Glycolysis:
The rate limiting or regulatory or key of glycolysis which undergo enzymes of glycolysis regulations are
- Hexokinase or Glucokinase
- Phosphofructokinase and
- Pyruvate Kinase enzymes
These are either induced (stimulated) or inhibited (suppressed) by different factors. This type of regulation is termed as allosteric regulation.
2. Tricarboxylic acid Cycle (TCA Cycle)
Definition:
- TCA cycle is defined as series of reactions where Acetyl Co-A is degraded to C*O_{2} with the production of Energy.
- This cyclic pathway also called as Citric acid cycle or Krebs' cycle, is the final common pathway for the oxidation of fuel molecules (such as carbohydrates, Proteins and fats).
Location: TCA cycle occurs in mitochondria (TCA cycle Enzymes are mitochondrial).
Importance/ Significance of TCA cycle:
- It produces energy in the form of ATP
- The intermediates formed in TCA cycle produce some amino acids, fatty acid, glucose etc.
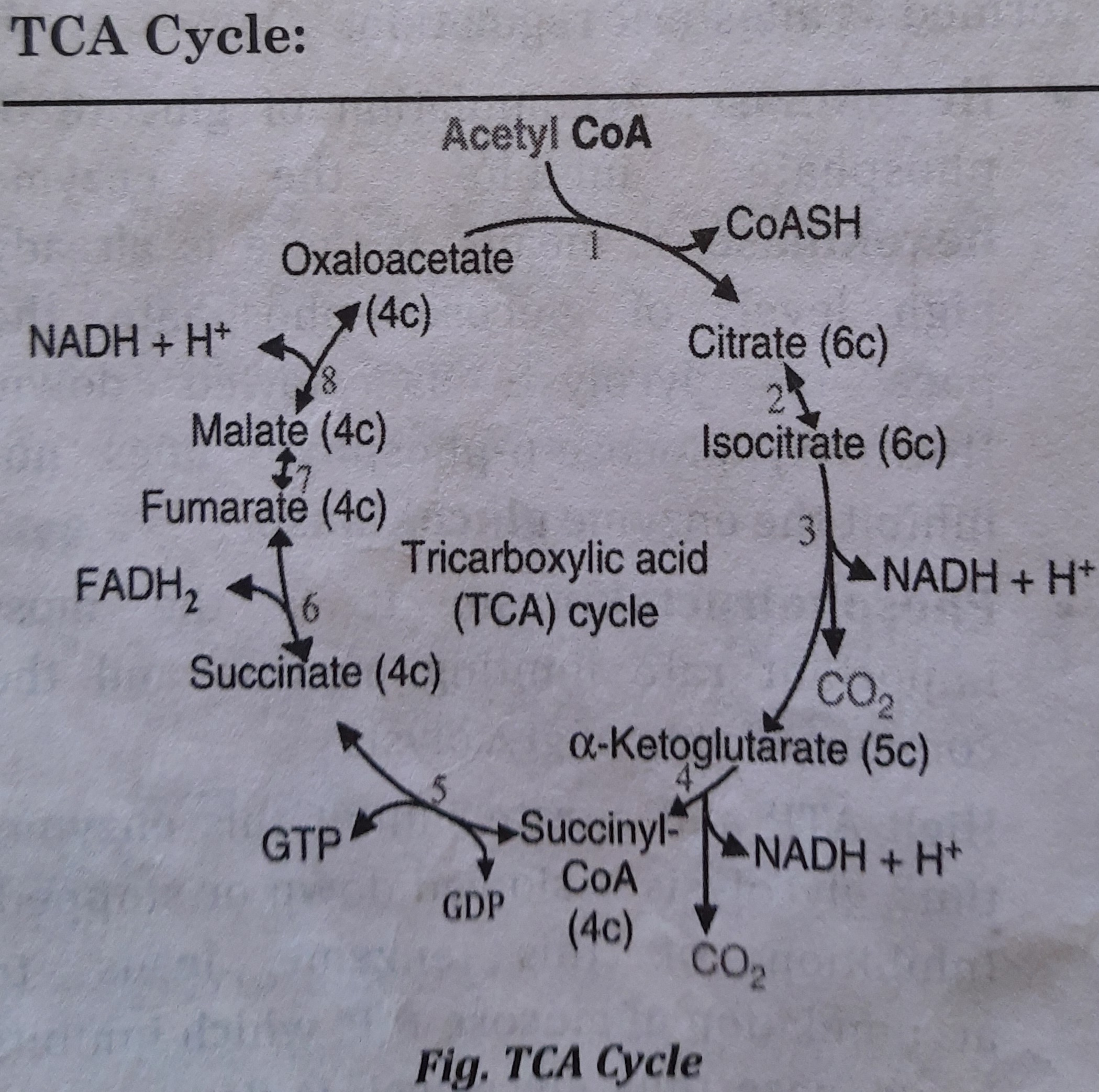
List of different enzymes involved in TCA Cycle
- Citrate synthase
- Aconitase
- Isocitrate dehydrogenase 4. Alpha-Ketoglutarate dehydrogenase
- Succinate thiokinase
- Succinate dehydrogenase 7. Fumarase
- Malate dehydrogenase
Reactions of the TCA Cycle: Reactions occur in following steps:
Preparation: Acetyl CoA is formed either from carbohydrates or proteins or fats. And oxaloacetate is initially formed from Pyruvate and then regenerated in TCA cycle. TCA cycle occurs on following steps -
- Step 1. Formation of citrate:
- Acetyl CoA is condensed with oxaloacetate to form citrate presence of citrate synthase in the
- Step 2. Formation of isocitrate:
- Citrate is isomerised to isocitrate by aconitase.
- Step 3. Formation of alpha ketoglutarate:
- Dehydrogenation and decarboxylation of Isocitrate forms alpha ketoglutarate. This reaction is catalyzed by isocitrate dehydrogenase.
- NADH is produced here which is oxidized in electron transport chain to generate three ATP.
- Step 4. Formation of succinyl coA:
- Dehydrogenation and decarboxylation of alpha keto glutarate forms succinyl CoA. This reaction is catalyzed by alpha ketoglutarate dehydrogenase.
- NADH is produced here which is oxidized in electron transport chain to generate three ATP.
- Step 5. Generation of succinate:
- Succinyl CoA forms succinate by succinate thiokinase. In this step ATP is generated.
- S 6. Formation of fumarate:
- Dehydrogenation of Succinate forms fumarate by succinate dehydrogenase.
- FADH2 is produced here which enters into electron transport chain to generate two ATP.
- Step 7. Formation of malate:
- Fumarate forms malate by fumarase.
- Step 8. Regeneration of oxaloacetate:
- Oxidation of malate forms oxaloacetate by malate dehydrogenase.
- NADH is produced here which is oxidized in electron transport chain to produce three ATP.
Energetics of TCA Cycle:
Energy produced from Acetyl CoA in TCA cycle
Enzymes |
Production of ATP |
Isocitrate dehydrogenase (1 NADH) |
3 ATP |
α-ketoglutarate dehydrogenase (1 NADH) |
3 ATP |
Succinate thiokinase |
1 ATP |
Succinate dehydrogenase (1 FADH2) |
2 ATP |
Malate dehydrogenase (1 NADH) |
3 ATP |
Total number of ATP produced |
12 ATPs |
Regulations of TCA Cycle:
Regulation of TCA Cycle occurs at
- Citrate synthase: ATP inactivates citrate synthase, resulting into inhibition of TCA Cycle.
- Isocitrate dehydrogenase: NADH inhibits but ADP activates this enzyme.
- Alpha keto Glutarate dehydrogenase: This enzyme is inhibited by NADH and Succinyl CoA. [Note: TCA cycle is inhibited by ATP and NADH
Inhibitors of TCA Cycle:
Some toxic or poisonous agents inhibit the enzymes thus, regulate the TCA Cycle
- Fluoroacetate: inhibits the Aconitase, thus, inhibits the TCA Cycle.
- Arsenite: inhibits the Alpha keto glutarate dehydrogenase, thus, inhibits the TCA Cycle.
- Malonate: inhibits the Succinate dehydrogenase, thus, inhibits the TCA cycle.
3. Glycogen Metabolism:
It includes both synthesis of glycogen (glycogenesis) as well as degradation of glycogen (glycogenolysis).
- Glycogenesis:
- Definition: Glycogenesis (Glycogen= a polysaccharide; genesis= synthesis) is defined as the synthesis of glycogen from glucose.
- Glycogen is a storage form carbohydrate in animal body. Approximately 400 gm of glycogen in muscle and 100 gm of glycogen is stored in liver cell in an adult human being.
- During well fed state, glycogen is formed in the body.
- Location: It occurs in the cytosol of liver and muscle cells.
- (Glucose)→→→ Glycogen
- Glycogenolysis
- Definition: Glycogenolysis (Glycogen a polysaccharide; lysis= breakdown) is defined as the breakdown of glycogen to form glucose.
- Glycogenolysis in liver forms glucose while glycogenolysis in musice finally forms glucose-6-phosphate which enter into glycolysis to produce ATP.
- Hepatic glycogenolysis (glycogenolysis that occur in liver) maintains glucose level in blood during fasting. After an 18 hours of fasting, the glycogen stored in liver is almost depleted.
- Location: It occurs in the cytosol of liver and muscle cells.
- Glycogen →→→ (Glucose)
Significance/ Functions of Glycogen:
Glycogen maintains blood glucose level and provides ATP (energy) for muscle contraction.
- When the glycogen stored in liver undergo degradation, it maintains or increases the blood glucose level.
- Similarly, when the glycogen stored in muscle undergo degradation, it provides ATP for muscle contraction. Muscle glycogen cannot maintain blood glucose level because phosphatase. muscle lacks the enzyme gluocse-6 phosphatase.
Regulations of Glycogen Metabolism:
- Glycogen metabolism is regualted by availability of substrates (glucose or glucose-6 phosphate) and by hormones (Insulin and glucagon etc)
Regulation of Glycogenesis:
- The regulatory or rate limiting enzyme for glycogenesis is Glycogen synthase which is activated in well fed state. It means, high substrates and high insulin activates the enzyme, thus, glycogen synthesis is stimulated. The counter regulatory hormones of Insulin, such as glucagon, adrenaline, etc. inhibits the enzyme glycogen synthase.
Regulation of Glycogenolysis:
- The regulatory enzyme for glycogenolysis is Glycogen phosphorylase which is activated during fasting. It means, low blood glucose and high glucagon and adrenaline activates the enzyme glycogen phosphorylase, thus, glycogen degradation is stimulated.
[Note: Glycogenesis is stimulated by insulin, under the influence of hyperglycemia, where as glycogenolysis is stimulated by glucagon, under the stimulus of hypoglycemia]
4. Gluconeogenesis
- Definition: Gluconeogenesis (Gluco= glucose; neo= new; genesis= synthesis) is defined as the synthesis of glucose from non-carbohydrate precursors.
- The non-carbohydrate compounds which participate in Gluconeogenesis are pyruvate, lactate, gluconeogenic amino acids, glycerol, propionyl CoA, etc.
- Location: Gluconeogenesis occurs partly in mitochondria and partly in cytosol of Liver, kidney, etc.
Significance of Gluconeogenesis:
Regulations of Gluconeogenesis:
The key gluconeogenic enzymes are
- Pyruvate carboxylase
- Phosphoenol pyruvate carboxykinase
- Fructose 1,6-bisphosphatase, and
- Glucose 6-phosphatase
High acetyl CoA, high citrate and high ATP activate Gluconeogenesis.
Hormones such as glucagon and glucocorticoids increase Gluconeogenesis, whereas, insulin inhibits the process. [Note: glucagon activates but insulin inhibits Gluconeogenesis.]
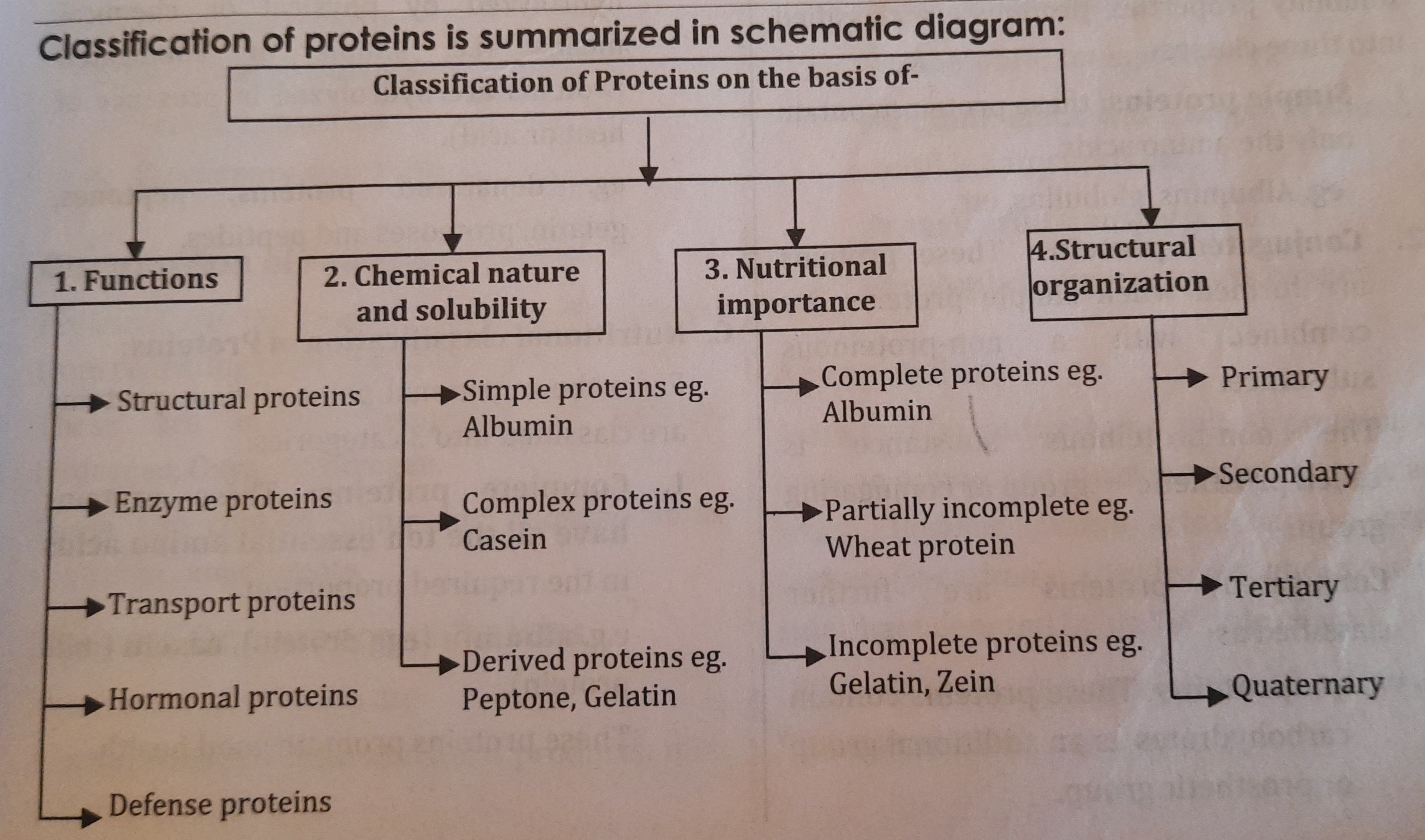
Proteins
Proteins are the most abundant organic molecules of living system.
The word protein is derived from Greek word, Proteios, which means holding the first place or primary, i.e Proteins are of primary importance for biological systems.
Definition
- Proteins are high molecular weight, nitrogen rich organic compounds which are of paramount importance for all living organisms.
- Proteins are polymer of amino acids, i.e. these are made up of repeating units of amino acids linked together through peptide bonds.
Classification of Proteins
Proteins have been classified on the basis of:
- Function
- Chemical nature and solubility properties
- Nutritional importance
- Structural organization
A. Classification of proteins on the basis of functions:
- On the basis of functions, proteins are classified into the following groups
- Structural proteins: eg. keratin of hair and nails, collagen..
- Enzymes or catalytic proteins: eg. hexokinase, pepsin
- Transport proteins: eg. hemoglobin, albumin.
- Hormonal proteins: eg. insulin, growth hormone.
- Contractile proteins: eg. actin, myosin.
- Genetic proteins: eg. nucleoproteins.
- Defense proteins: eg. immunoglobulins, snake venoms.
B. Classification of proteins on the basis of chemical nature and solubility properties:
On the basis of chemical nature and solubility properties, proteins are classified into three classes
- Simple proteins:
- These proteins contain only the amino acids. eg. Albumins, globulins, etc.
- Conjugated proteins:
- These proteins are formed when simple proteins are combined with non-proteinous a substance. The non-proteinous substance called prosthetic group or conjugating group.
Conjugated proteins are further classified as-
- Glycoproteins: These proteins contain carbohydrates as an additional group or prosthetic group. eg. immunoglobulins (antibodies), blood group antigens, etc.
- Lipoproteins: These proteins contain lipid as an additional group. eg. Serum lipoproteins such as LDL (Low density lipoprotein), HDL (High density lipoprotein), etc.
- Phosphoproteins: These proteins contain phosphorus as an additional group. eg. Casein a phospho-protein present in milk.
- Metalloproteins: These contain metal ions. eg. Hemoglobin is a protein which contains iron.
- Derived proteins: These are formed when simple or conjugated proteins are hydrolyzed by physical or chemical means (i.e. simple or conjugated proteins are hydrolyzed in presence of heat or acid). eg. denatured proteins, peptones, gelatin, proteoses and peptides.
C. Nutritional classification of Proteins:
From the nutritional point of view, proteins are classified into 3 categories:
- Complete proteins:
- These proteins have all the ten essential amino acids in the required proportion. e.g. albumin (egg protein), casein (milk protein).
- These proteins promote good health.
- Partially incomplete proteins:
- These proteins are partially lacking one or more essential amino acids. eg wheat and rice proteins. These proteins limit some of the essential amino acids such as Lysine and Threonine.
- Hence,they can promote moderate growth.
- Incomplete proteins:
- These proteins completely lack one or more essential amino acids (ie. some amino acids are absent) e.g. Gelatin and zein protein (maize protein). Gelatin do not have Tryptophan and Lysine.
- Hence they do not promote growth at all.
D. Classification of proteins on the basis of Structural organization:
On the basis of structural organization, proteins are classified as
- Primary structure
- Secondary structure
- Tertiary structure
- Quaternary structure
Composition of Proteins
- Proteins are the organic compounds formed from repeating units of amino acids.
- These are mainly composed of Carbon, Hydrogen, Oxygen, Nitrogen.
- These also contain Sulfur and Phosphorus as the minor constituents.
Sources/ Occurrence of Proteins
The sources of proteins are:
- Animal sources: Meat and meat products; milk and milk products etc.
- Vegetable sources: Legumes, pulses, cereals etc.
Functions of Proteins
Proteins perform varieties of functions
- Proteins are body building food. They provide energy. One gram of protein yields 4kcal energy.
- Proteins are the component of cell membrane such as glycoprotein, ankyrin, spectrin.
- Proteins are responsible for strength and structure of body. eg. collagen and elastin. • Proteins act as biological catalyst called as enzymes. eg. amylase, trypsin etc.
- Proteins act as hormone. Most of the hormones are made up of proteins and amino acids. eg. insulin, growth hormone, thyroid hormones, oxytocin, etc.
- Antibodies are protein in nature. They serve as first line of defense against invading pathogens.
- Proteins act as transport media for a number of substances
- Hemoglobin: transports oxygen.
- Albumin: binds and transports bilirubin, steroids, fatty acids etc.
- Transferrin: transports iron.
- Ceruloplasmin: transports copper.
Amino Acids
Amino acids are the basic unit of proteins that have an amino and a carboxyl (carboxylic acid) group. Besides, amino acids have a central carbon (a-carbon), a hydrogen and a variable side chain denoted by an "R" side chain.
"R" is different for each of the 20 amino acids found in protein.
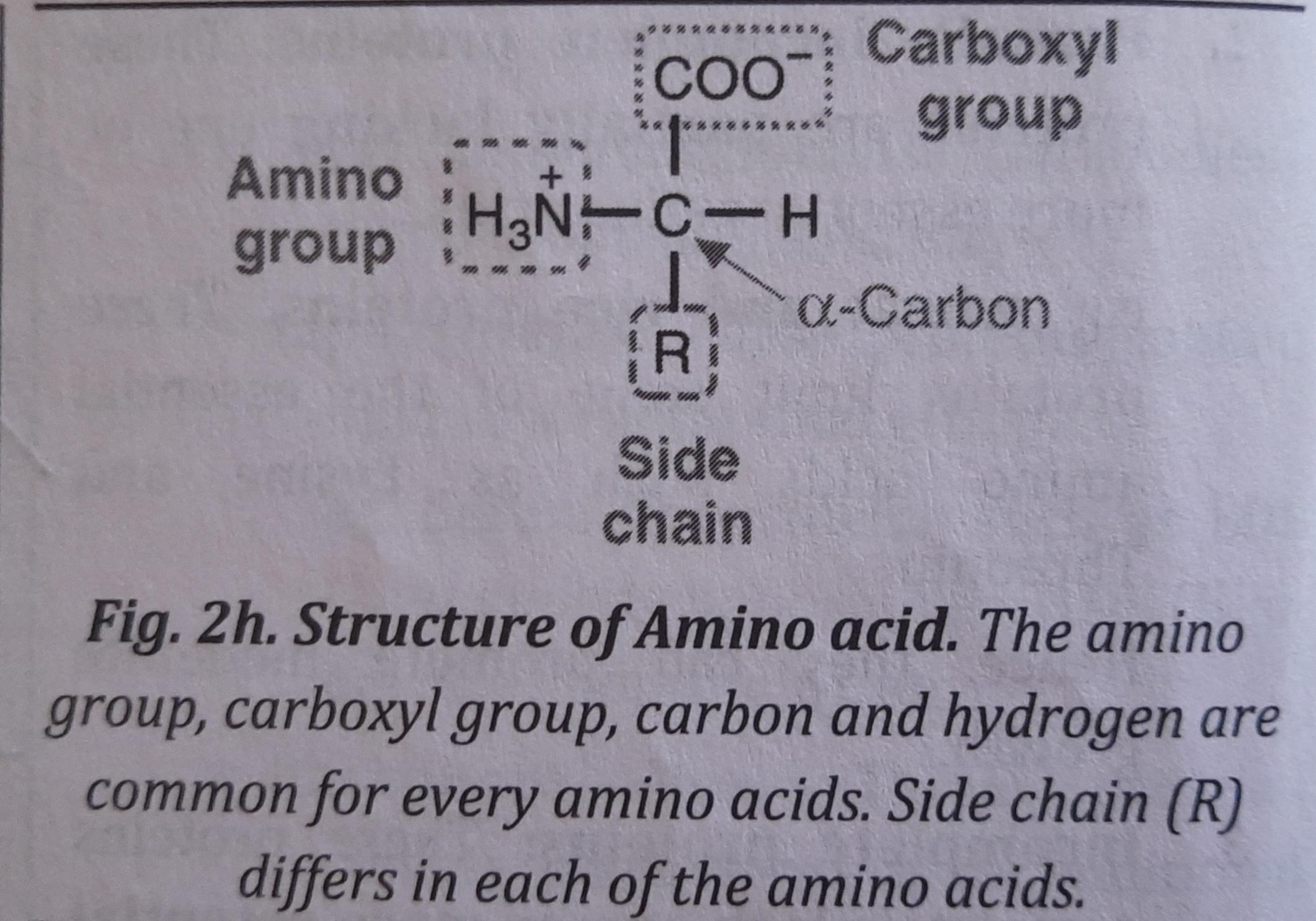
Classification of Amino acids
Amino acids are classified into various classes on the basis of their:
- Nutritional importance
- Metabolic fate
- Chemical nature of side chain
A. On the basis of nutritional importance, amino acids are classified as:
- Essential Amino acids:
- Those amino acids which cannot be synthesized by body hence, must be taken in diet are known as dietary essential amino acids.
- These are required for proper growth and maintenance of individual. eg. Phenylalanine, Valine, Threonine, Tryptophan, Isoleucin, Methionine, Histidine, Arginine, Leucine, Lysine.
- [Abbreviation: PVT TIM HALL].
- (Histidine and Arginine may be grouped as semi-essential amino acids)
- Semi-essential Amino acids:
- These amino acids are essential for children so must be taken in diet. However, adults can synthesize these amino acids, so, need not be consumed in diet. Therefore, these amino acids are termed as semi-essential amino acids. eg. Histidine and Arginine.
- Non-essential amino acids:
- Those amino acids which can be synthesized by body, hence, need not be consumed in the diet are known as dietary non-essential amino acids. These are also required for growth and maintenance of individual. eg. Alanine, Asparagine, Aspartic acid, Cysteine, Glutamic acid, Glutamine, Glycine, Proline, Serine, Tyrosine.
- [Note: Both the essential and non-essential amino acids are required for proper body functions. These differ only in the context of whether these should be consumed in diet or can be synthesized in body.]
B. On the basis of metabolic fate:
The carbon skeleton of amino acids can synthesize either glucose or fat or both- glucose as well as fat, accordingly amino acids are classified as:
- Glucogenic or Glycogenic Amino acids:
- These amino acids can form glucose or glycogen, hence, known as glucogenic or glycogenic amino acids. eg.- Ala, Asp, Gly, Met etc. Glucogenic amino acids can synthesize either glucose or any intermediates of TCA cycle.
- Ketogenic Amino acids:
- These amino acids can synthesize fat or ketone bodies. eg.- Leu, Lys. These amino acids can form acetyl CoA or acetoacetyl CoA or acetoacetate.
- Glycogenic and Ketogenic both:
- These amino acids can synthesize both glucose as well as fat or ketone bodies. eg.- Phe, Trp, Tyr and Ile.
C. On the basis of chemical nature of side chain: On the basis of chemical nature of side chain, amino acids are classified as
- Amino acids with aliphatic side chain: eg. Gly, Ala
- Amino acids with aromatic side chain: eg. Phe, Tyr, Trp
- Amino acids containing hydroxyl group: eg. Ser, Thr
- Sulfur containing amino acids: eg. Cys, Met
- Acidic amino acids and their amides: eg. Asp, Asn, Glu and Gln
- Basic amino acids: eg.His, Arg and Lys
- Imino acid: eg. Pro
Functions of Amino acids
Amino acids perform various functions
- Glucogenic amino acids synthesize glucose through gluconeogenesis.
- Amino acids can synthesize some specialized products such as:
- Tyrosine: synthesizes thyroid hormones which are required for general metabolism in the body.
- Tryptophan: synthesizes serotonin which is a stimulator of brain activity.
- Arginine: can form Nitric oxide which is a vasodilator, relaxes smooth muscle and reduces blood pressure.
- Glutamate: can synthesize gamma amino butyric acid (GABA), which is an inhibitory neurotransmitter.
- Amino acids form protein. Thus, functions of amino acids correspond to the functions of proteins, such as catalytic functions, hormonal functions, defensive functions, etc.
Peptides
When a number of amino acids are linked by peptide bonds, a polypeptide or protein is formed.
Dipeptides have two amino acids, tripeptides have three amino acids and so on.
- Dipeptide: two amino acids are linked by a peptide bond to form dipeptide. eg. Aspartame: it is an artificial sweetener formed from Aspartic acid and Phenylalanine. Formation of dipeptide is shown in figure 2i.
- Tripeptide: three amino acids are linked by two peptide bond to form tripeptide. eg. Glutathione, Thyrotropin releasing hormone, etc. Glutathione: It is a tripeptide formed from glutamate, cysteine and glycine. It is an antioxidant in animal. Thyrotropin releasing hormone (TRH): It is a tripeptide formed from glutamate, histidine and proline. It stimulates the release of thyroid stimulating hormone from anterior pituitary gland.
- Polypetide: many amino acids are linked by peptide bonds to form a polypeptide or protein. eg. Insulin is made up of 51 amino acids. It is a polypeptide hormone which is required for glucose homeostasis.
Amino acid metabolism is discussed under different stages with following reactions:
- Transamination:
- It is defined as transfer of an amino group from an amino acid to a keto acid to form a new amino acid and a new keto acid. Enzyme used for transamination reaction is-Aminotransferase (Transaminase).
- Transamination reactions can synthesize non essential amino acids like Alanine, Glutamate, Aspartate, etc.
- During the transamination reaction, most often, glutamate is formed which can undergo oxidative deamination.
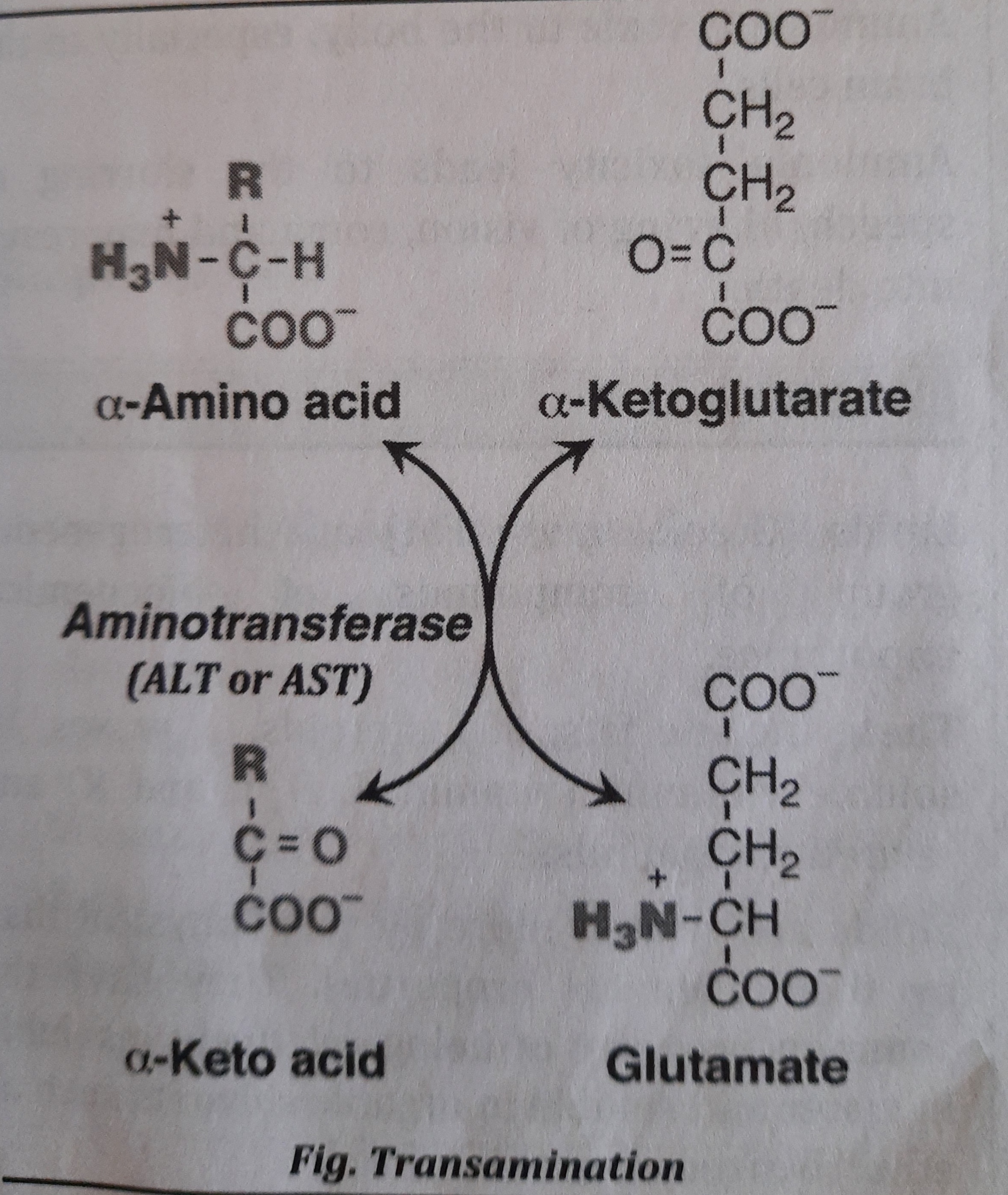
- Deamination (de= removal):
- It is a process of removal of Amino group (as N*H_{3} ] which is used for the synthesis of urea in liver cell.
- Enzyme involved during deamination is Glutamate dehydrogenase (GDH)
- After removing amino group, the amino acid becomes ketoacid (Alpha ketoglutaric acid, a-KG) which can synthesize glucose or give energy.
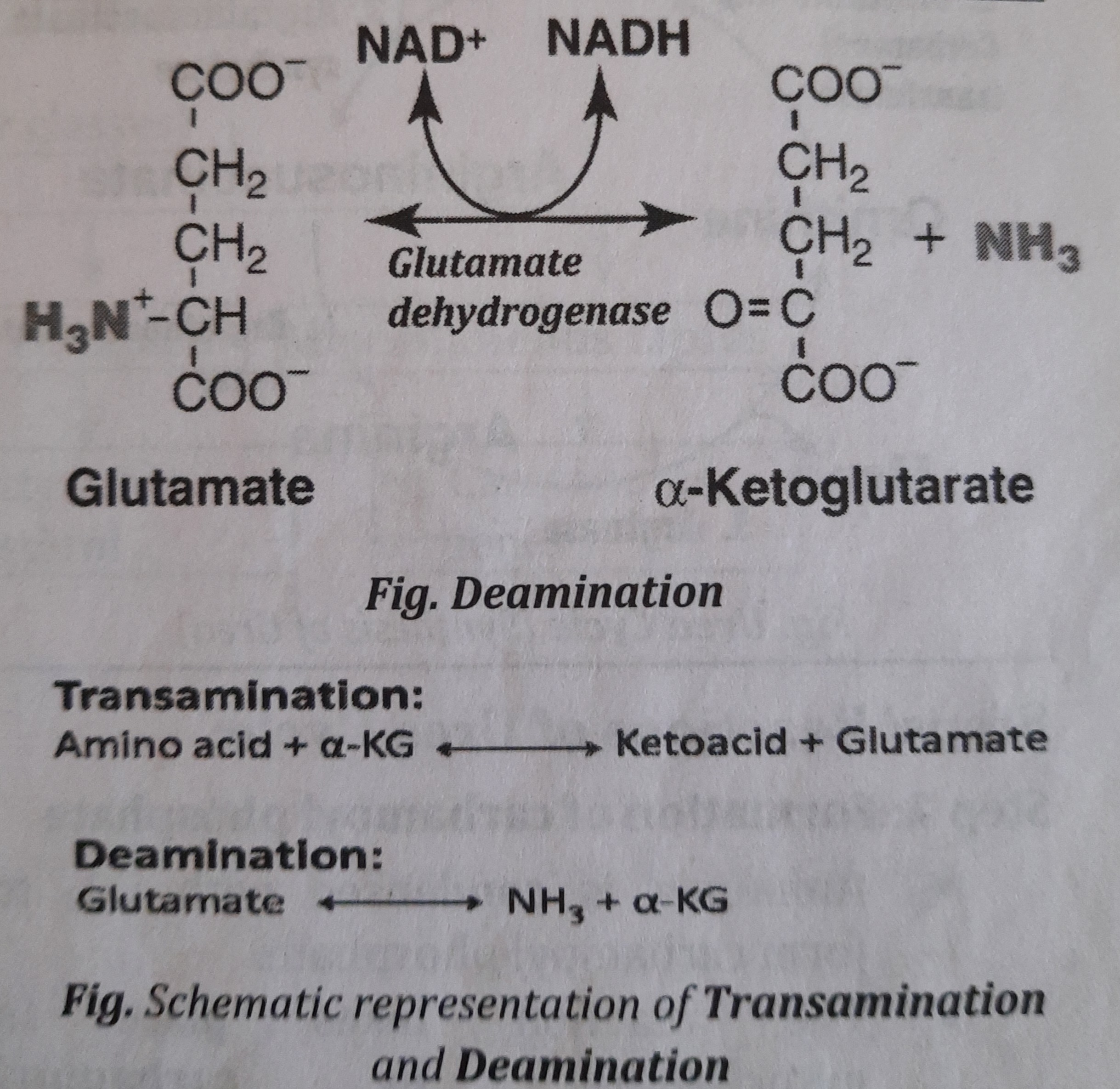
- Synthesis of Urea or Urea Cycle
- After transamination followed by deamination, the released ammonia (N*H_{3}) is toxic to the body especially to the brain cells. So, to reduce the toxicity of ammonia, hepatic cells convert it into urea which is the less toxic form. It means, synthesis of urea is to prevent body from the toxicity of N*H_{3}
- Due to the presence of Arginase, urea is synthesized only in Liver. Thus, produced urea is released into blood and cleared by the kidneys.
- Urea cycle is also called Krebs Hansleit Cycle or Ornithine Cycle. It is the first metabolic cycle to be discovered.
- Location: Synthesis of urea takes place partly in mitochondria and partly in cytosol of liver cells.
Synthesis of urea can be described in five steps. Steps one and two occur inside mitochondria and the rest of the steps on the cytosol.
Steps/Reactions of Urea Cycle:
- Step 1: Formation of carbamoyl phosphate
- Ammonia is condensed with C*O_{2} to form carbamoyl phosphate.
- The reaction takes place in mitochondria by carbamoyl phosphate synthetase I (CPS I). This enzyme is activated by N-acetylglutamate (NAG).
- This reaction is the rate limiting step in urea cycle.
- Step 2: Formation of citrulline
- The carbamoyl group of carbamoyl phosphate is transferred to the ornithine to form citrulline. This reaction also takes place in mitochondria by ornithine carbamoyl transferase.
- The citrulline comes to the cytosol where the further reactions take place.
- Step 3: Formation of Argininosuccinate
- One moleculeof aspartic acid is added to citrulline forming argininosuccinate in the presence of argininosuccinate synthetase.
- Step 4: Formation of Arginine
- Arginiosuccinate is cleaved by form
- The fumarate formed may be funneled into TCA cycle. argininosuccinate lyase to arginine and fumarate.
- Step 5: Formation of Urea
- Arginine is hydrolyzed to urea and ornithine.
- Enzyme used is Arginase, which is present only in liver.
- The ornithine returns to the mitochondria for another cycle of reaction.
Regulations of Urea Cycle
The major regulatory enzyme of urea cycle is carbamoyl phosphate synthetase-1 (CPS-I) which is activated by N-acetyl Glutamate (NAG). In general, urea cycle enzymes are elevated with increased dietary intake of protein and during starvation.
Disorders of Urea Cycle
- Defect in any one of the enzymes involved in urea synthesis results into hyperammonemia (high levels of ammonia in blood).
- Ammonia is toxic to the body, especially to the brain cells.
- Ammonia toxicity leads to the slurring of speech, blurring of vision, coma and may result into death.
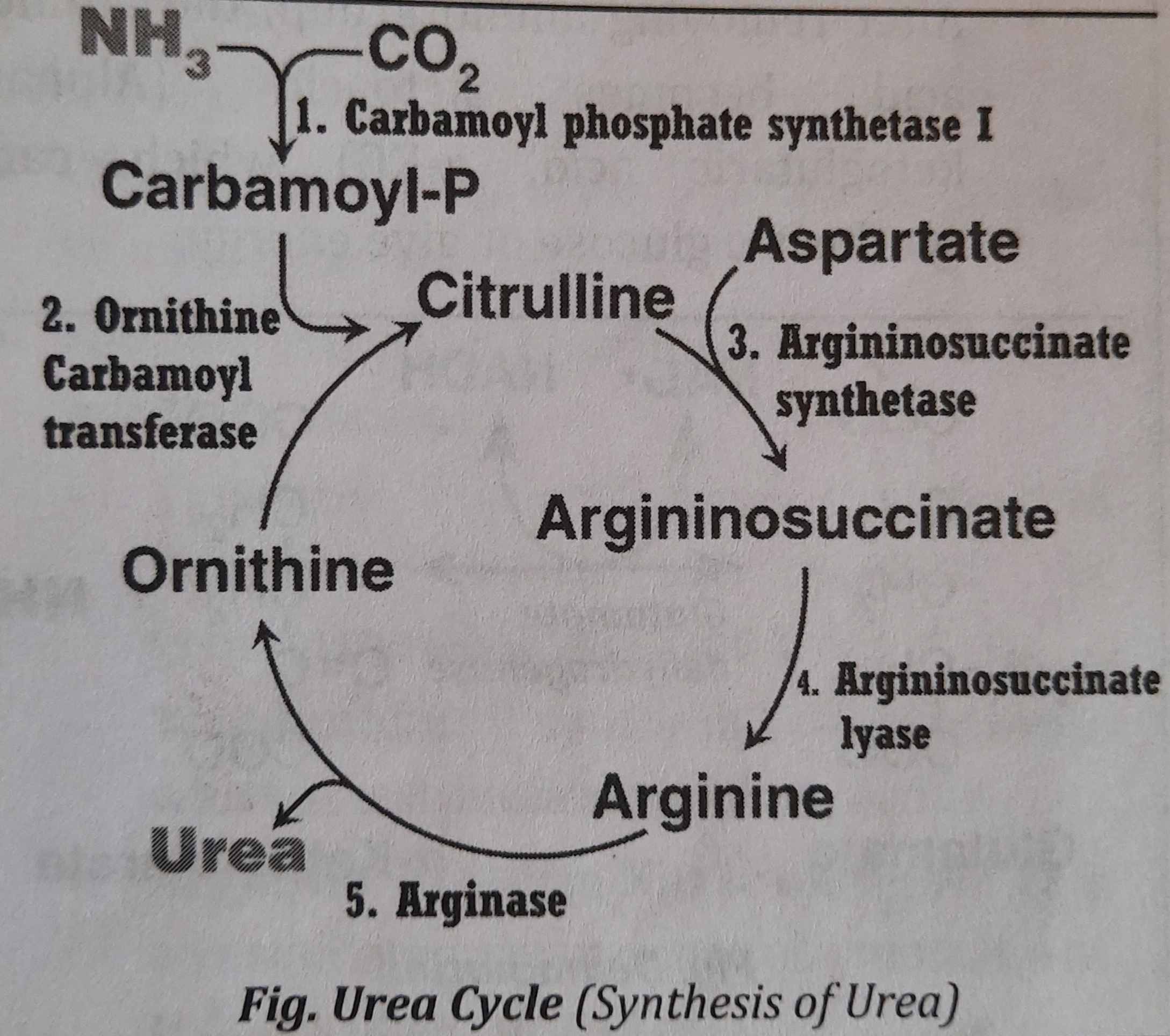
Lipids
- Lipids (Greek, lipos= Fat) are heterogeneous group of compounds of biochemical importance.
- These include fats, oils, steroids, soluble vitamins (vitamin A, D, E and K) and related compounds.
- Lipids are related more by their physical than by their chemical properties. They have the common property of being relatively insoluble in water and soluble in organic solvents such as ether, benzene and chloroform.
Definition
- Lipids are heterogeneous group of organic compounds which are insoluble in water but soluble in organic solvents like benzene, ether, chloroform etc.
- Lipids are consumable by the living organisms. When lipids are metabolized, they release large amounts of energy and thus are useful to living organisms. Examples of lipids: Oil, Fats, Triglyceride (TG), Cholesterol etc.
Classification of Lipids
On the basis of chemical nature, lipids are classified as:
- Simple lipid
- Complex lipid
- Derived lipid
- Miscellaneous lipid nomble
_1668058172.jpg)
- Simple Lipids
- Simple lipids are the esters of fatty acids with alcohols. These are of two types:
- Fats and oils or Triglyceride (TG): These have fatty acids attached with glycerol. TG is also known as TAG (triacyl glycerol), the structure of which is shown in figure 2j.
- Waxes: These have fatty acids attached with higher molecular weight alcohol (cetyl alcohol).
- Complex lipids
- Complex lipids contain fatty acids attached with alcohol and an additional groups such as either phosphate or carbohydrates or proteins etc. eg. Phospholipids, Glycolipids, Lipoproteins etc.
- Phospholipids: These lipids contain fatty acids and alcohol (glycerol or sphingosine) in addition to phosphoric acid and nitrogen bases. eg. Lecithin (Phosphatidyl cholin), Cephalin (Phosphatidyl ethanolamine), Cardiolipin etc.
- Glycolipids: These lipids contain fatty acids and alcohol (sphingol or sphingosine). These also contain carbohydrate as the additional group. eg. cerebrosides, gangliosides etc.
- Lipoproteins: These are the macromolecular complexes formed from lipids and proteins. Lipoproteins help transport lipids in blood. eg. chylomicron, VLDL (Very low density lipoprotein), LDL (Low density lipoprotein), HDL (High density lipoprotein).
- Derived lipids
- These are the compounds obtained from hydrolysis of simple lipids and complex lipids. eg. Fatty acids, Glycerol, Cholesterol,
- Hydrolysis of TG (breakdown of TG), form three fatty acids and glycerol.
- Miscellaneous Lipids:
- These include a large number of compounds having characteristics of lipids. eg. Carotenoids, squalene, terpenes, lipid soluble vitamins, such as vitamins A, D, E and K, etc.
Fatty Acids
- Fatty acids are the components of TG. These are the building blocks for most of the lipids.
- Fatty acids are the carboxylic acids with hydrocarbon tail. The carboxylic acid portion is polar (water soluble), where long as hydrocarbon chain is non-polar. Hence, because of having both polar and non-polar regions, fatty acids are called as amphipathic molecules.
- Eg. Acetic acid, Palmitic acid, Stearic acid, Linoleic acid, Linolenic acid and Arachidonic acid, etc.
Classification of Fatty acids:
Fatty acids are classified on the basis of
- Nutritional requirement
- Nature of chain
- Chain length
1. On the basis of nutritional requirement, fatty acids are classified as:
- Essential fatty acids:
- These fatty acids cannot be synthesized by body and therefore they should be taken in diet. e.g. Linoleic acid, Linolenic acid and Arachidonic acid.
- Non-essential fatty acids:
- These fatty acids can be synthesized in body and therefore, need not to be supplied in diet. e.g. Acetic acid, Palmitic acid and Stearic acid.
2. On the basis of nature of chain, fatty acids are classified as:
- Saturated fatty acids: Fatty acids having only single bond on their structure are called as Saturated fatty acids. eg. Acetic acid, Palmitic acid and Stearic acid
- Unsaturated fatty acids: Fatty acids having double or triple bond on their structure are called as unsaturated fatty acids. eg. Linoleic acid, Linolenic acid and Arachidonic acid.
3. On the basis of chain length, fatty acids are classified as:
- Short chain fatty acids: Fatty acids having upto six carbons are called as short chain fatty acids. eg. Propionic acid, Butyric acid etc.
- Medium chain fatty acids: Fatty acids having eight to 14 carbons are called as medium chain fatty acids. eg. Capric acid, Myristic acid, etc.
- Long chain fatty acids: Fatty acids e having 16 to 24 carbons are called as a long chain fatty acids. eg. Palmitic acid, Stearic acid, etc.
Functions of Fatty acids:
- Hormone synthesis: Dietary essential fatty acid, such as Arachidonic acid synthesizes prostaglandin, prostacyclin, etc. which are the local hormones.
- Prostaglandin, such as, thromboxane (TXA2) has a role in vasoconstriction and platelets aggregation.
- Prostacyclin (PGI2) has the major role in vasodilatation and inhibition of platelet aggregation.
- Membrane lipids: Essential fatty acids are used as membrane components and structural elements of tissues.
- Storage molecule: Fatty acids, such as Palmitic acids are the components of stored fat in form of TG.
- Fuel molecule: Fatty acids produce a huge amount of energy when these undergo oxidation.
The energy produced after complete oxidation of one molecule of Palmitic acid is 129 ATP (Adenosine triphosphate).
Sources (Occurrence) of Fatty acids:
Fatty acids are present in plant and animal sources. They mainly occur in the esterified form as major constituents of various lipids.
- Palmitic acid and Stearic acid are present in body fat.
- Capric acid and Myristic acid are present in butter and coconut oil.
- Linoleic acid, Linolenic acid and Arachidonic acid are present in vegetable oils.
Composition of Lipids
Lipids are the organic compounds which are composed of carbon, hydrogen, oxygen. Besides, the complex lipids also contain carbohydrate, phosphorus, proteins etc. Most of the lipids contain fatty acid on their structure.
Sources of Lipids
- Plant sources: Vegetable oil such as mustard, sesame, soybean, sunflower and coconut oil etc.
- Animal sources: Butter, ghee, animal fat (TG). egg yolk etc.
Function (Importance) of lipids
- Lipids are the(concentrated source of energy. One gram of lipid provides 9kcal energy.
- Lipids (brown adipose fats) produces heat. Lipids also serve as the thermal insulator against changes in external temperature.
- Lipids protect internal organs by providing acushioning effect (pads of fat). These also give shape to the body.
- Lipids, such as glycolipids, phospholipids and cholesterol are the constituent of membrane and regulate the membrane permeability.
- Lipids eg. Cholesterol synthesize bile acids, vitamin D, steroid hormones etc. Lipids improve taste and palatability of foods.
- Lipoproteins act as transport vehicle, these help in transport of cholesterol, triglycerides etc.
- Lipids, such as Linoleic, Linolenic and Arachidonic acid help in reducing the incidence of coronary heart disease (CHD), atherosclerosis etc.
- Arachidonic acid is involved in the synthesis of prostaglandin. The major effects of the prostaglandin (such as thromboxane A2; TXA2) are vasoconstriction and platelet aggregation.
Fatty acid oxidation:
The B-oxidation pathway is primarily used by the body to oxidize fatty acids. The oxidation of fatty acids at carbon is what it is referred to as. With the loss of two carbon atoms in the form of Acetyl CoA at a time, this mechanism causes a fatty acid to cleave between a and a carbon atom.
In the mitochondria of liver or muscle cells, fatty acids are oxidized. Eight Acetyl CoA are produced when a palmitic acid (a fatty acid with 16 carbons) is oxidized, and these Acetyl CoA are mostly oxidized in the TCA cycle to produce ATP. The production of ketone bodies or cholesterol uses acetyl CoA as a substrate.
One fatty acid molecule, such as palmitic acid, can be completely oxidized to yield 129 ATP in total.
Different stages of Fatty acid oxidation:
- Activation of Fatty acids: Fatty acid is condensed with Coenzyme A (CoA or CoA SH) with the use of ATP in cytosol to form Acyl Co-A which is the activated form of fatty acid. Enzyme used during the process is Acyl CoA synthetase or Thiokinase.
- Transport of Acyl Co-A into the Mitochondria: Since, the fatty acid oxidizing enzymes are present in mitochondria. So, the Acyl Co-A is transported inside the mitochondria with the help of carnitine shuttle. Now, inside the mitochondria, Acyl CoA is ready for oxidation.
- Proper ẞ-oxidation inside mitochondria: Acyl CoA is oxidized inside the mitochondria in different steps which are summarized below.
- Step 1: Oxidation:
- Acyl CoA is oxidized to form a 42-trans Enoyl CoA by Acyl CoA dehydrogenase which is FAD dependent.
- During the process, FAD is reduced to FADH2
- One FADH2 produces two ATP when oxidized in electron transport chain in mitochondria.
- Step 2: Hydration:
- The A2-trans-Enoyl CoA forms ẞ-hydroxy acyl CoA by the enzyme enoyl CoA hydratase.
- Step 3: Oxidation:
- The B-hydroxy acyl CoA is again oxidized to form B-keto acyl CoA by hydroxyacyl COA dehydrogenase which is NAD+ dependent.
- During this oxidation, NAD is reduced to NADH.
- One NADH produces 3 ATP when oxidized in electron transport chain in mitochondria.
- Step 4: Cleavage:
- B-keto acyl CoA is splitted into an Acetyl CoA and a molecule of Acyl CoA, which is a two carbon shorter than the original reacting fatty acid.
- The newly formed Acyl CoA (with two carbon less) will sequentially undergo further cycles of step 1,2,3 and 4 of beta oxidation until the fatty acid is completely converted to Acetyl CoA.
- Finally, complete oxidation of one palmitic acid (16 Carbon containing fatty acid) will produce eight Acetyl CoA.
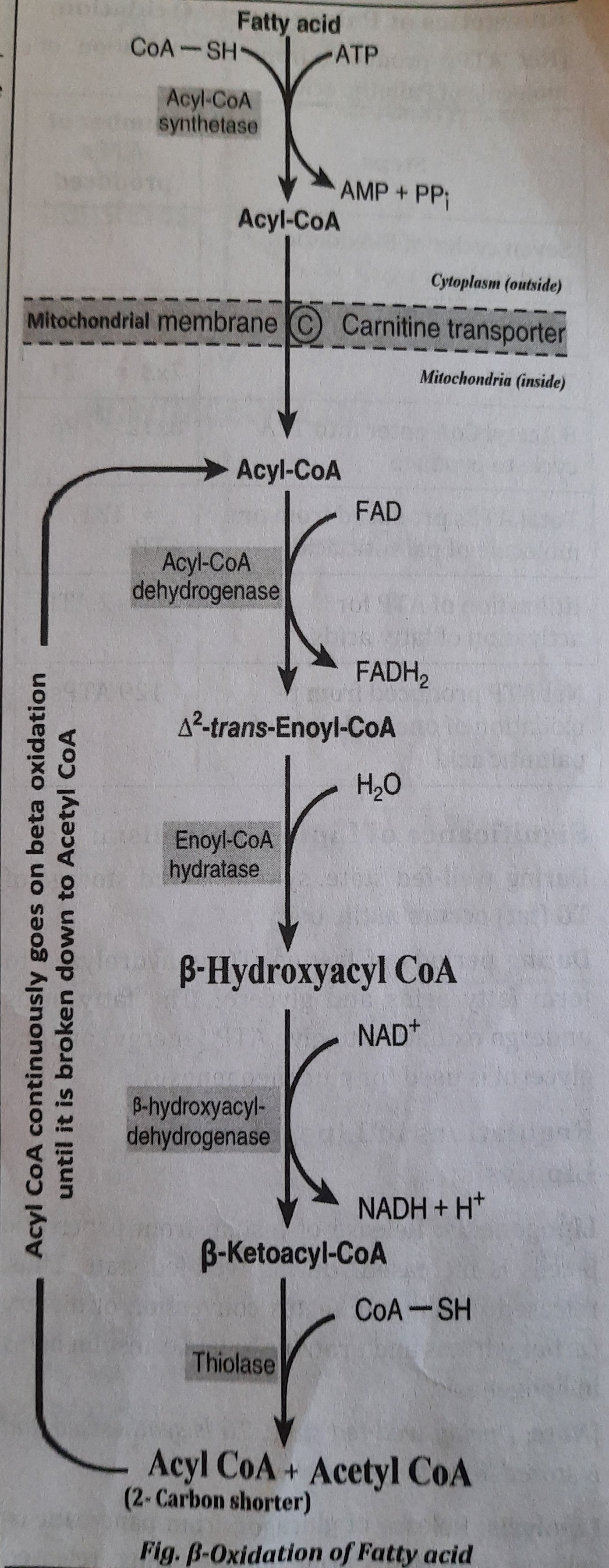
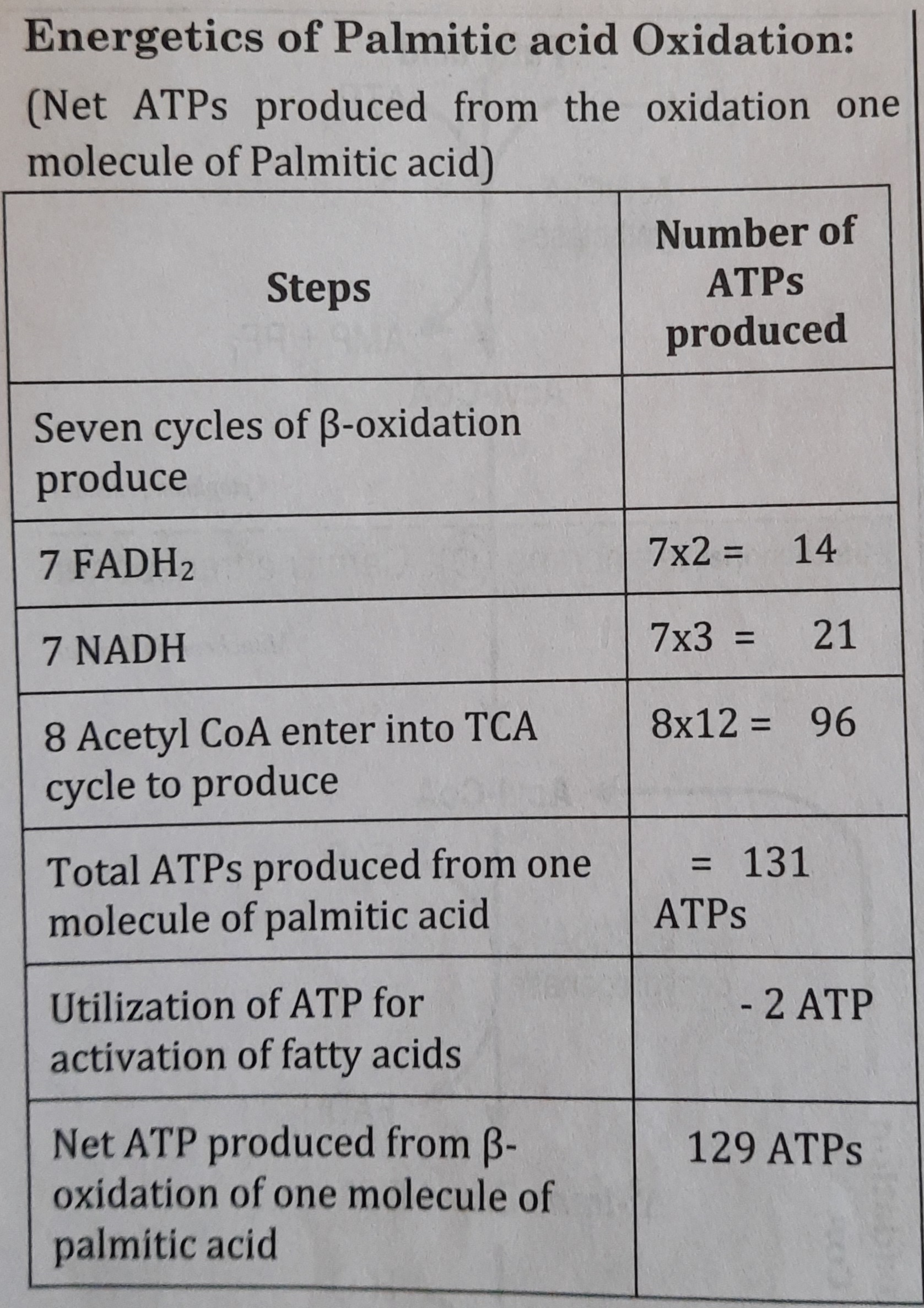